Summary
This paper describes the interaction betweenhydraulic fractures and the multi-porosity system of shale reservoirs. During the process of hydraulic fracturing, a complex fracture network consisting of primary and secondary fractures is created. It is postulated that only shale porosities that are in contact with these hydraulic fractures will contribute to hydrocarbon production. Furthermore, we propose a way to maximise well productivity by injecting micro-sized proppants into the secondary hydraulic fractures and reactivated natural fractures to prevent them from closing during production and thereby extending the fracture network. Both laboratory experiments and field tests have shown encouraging results of using micro-sized proppants to enhance the productivity of Barnett shale. More research is warranted to study the applicability and optimisation of micro-sized proppants in production enhancement in other shale formations.
Key words: Shale reservoir, micro-sized proppant, maximizing production, natural fracture, hydraulic fracture.
1. Introduction
The shale revolution in North America in the last decade has captured the attention of both researchers and practitioners in the oil and gas industry. The current research & development effort focuses on the optimisation of multi-stage hydraulic fracturing in long horizontal wells inshalereservoirs. However, afundamentalunderstanding of the interaction between hydraulic fractures, natural fractures and the pore system of the shale reservoir is lacking. The objective of this paper is to fill this knowledge gap. Based on this understanding, we propose a way to maximise productivity of shale reservoirs by increasing the connectivity between the hydraulic fractures and the pore system of the reservoir.
2. Pore system in shale reservoirs
The pore structure of a shale reservoir is more complicated than that of a conventional reservoir because shale contains both organic matter and inorganic matter. The former consists of kerogen and the latter carbonate, quartz, feldspar and clays. The various types of natural porosities of a shale formation can be classified as Figure 1.
- Organic pores
Organic matter such as kerogen tends to develop intraparticle pores during the evolution and expulsion of hydrocarbons from the kerogen [1]. These intraparticle pores have been observed in all mudstones, and believed to contribute dominantly to the total porosity. Their shapes can be semispherical, bubble-like, or pendular. Organic porosity can also be enhanced by cracks or fractures within the organic matter.
- Inorganic pores
Inorganic pores are those that exist in inorganic minerals such as clays, quartz and feldspar. Microscopic observations show that they exist as partially open floccules, triangular pores at clay platelet junctions or lenticular, slit-like pores between clay flakes. These inorganic pores may reduce in size during hydrocarbon production due to compression of the slit-like pore structures because of the increase in effective vertical stress [1].
Figure 1. Porosity system in shale reservoirs.
- Pore channels
Pore channels are interparticle pores. They form connections among organic pores, inorganic pores, and between them. They exist in inorganic matter as triangular, slit-shaped pores or vuggy pores [1].
- Natural fractures
Thermally mature shales usually contain vertical, sub vertical and in some cases horizontal natural fractures. These fractures are usually filled with mineral precipitates, such as calcite, dolomite, pyrite, barite and clays. In some cases, non-mineralised natural fractures may contribute to substantial gas storage capacity as observed in the Bakken shale [1].
3. Gas storage in shale reservoirs
Natural gas can be stored in any of the aforementioned natural porosities of a shale reservoir. It can exist as free gas in the pore space, absorbed gas on the surfaces of the organic and inorganic pores and on the wall of the natural fractures. Furthermore, it can be stored as dissolved gas in the kerogen. During production from shale reservoirs, free gas is produced first. The initial production and subsequent rapid decline in gas rate are both due to free gas production. This decline rate is hyperbolic. Only later is the absorbed gas produced and the decline rate is exponential [2]. Gas absorbed on the surface of the pore system can be 6 - 8 times the amount of free gas [3]. Due to its slow diffusion rate from the kerogen to the pore system, dissolved gas contributes insignificantly to hydrocarbon production.
The relative amount of gas stored in the organic pores, inorganic pores, pore channels and natural fractures varies from shale to shale. Usually natural fractures do not exhibit significant gas storage capacity, most often at best 0.5% [3]. However, in the highly fractured Bakken shale, a significant amount of gas comes from the non-mineralised natural fractures [1].
4. Permeability in shale reservoirs
Permeability is due to both pore size and interconnectivity between pores. The pore size in shale reservoirs is very small. Figure 2 shows the pore size distribution in various North American shales [4]. It ranges from less than 1 to 200nm. An examination of pore size distribution of global unconventional shale reservoirs by Zou also concludes that it varies from about 5 - 200nm [5]. A study by Nelson concludes that the pore-throat size distribution in shale reservoirs ranges from several to hundreds of nm [6]. In addition, the pores within a shale reservoir are usually poorly connected. Both the small pore size and the poor connectivity between pores lead to the extremely low permeability of shale reservoirs (< 0.001 - 0.01mD).
Figure 2 . Pore size distribution of North American shales [4].
5. Gas transport in shale reservoirs
Gas transport in a shale reservoir is different from that in a conventional reservoir due to the very small pores. As the pore size shrinks, the mean free path of gas molecule is comparable to that of a pore or pore channel. Under this condition, Darcy’s law does not apply. Other flow mechanisms such as slip flow, surface diffusion, Knudsen diffusion, Langmuir desorption, and molecular diffusion come into play. These flow mechanisms are usually much slower than Darcy’s flow. Hence, in the absence of reservoir stimulation by hydraulic fracturing, there is practically no production from shale reservoirs.
6. Interaction between horizontal well, hydraulic frac- tures and shale porosities
In conventional reservoirs, a horizontal well usually has a production rate several times that of a vertical well due to larger contact area between the well and the reservoir. However, in a shale reservoir, a horizontal well by itself does not lead to increase in hydrocarbon production due to the very low reservoir permeability. The function of a horizontal well, however, is to allow multiple hydraulic fractures to be placed along its length. It is the hydraulic fractures that create the connection between the well and the reservoir thus allowing flow of gas into the well. Each stage of hydraulic fracture increases the contact between the well and the reservoir. Therefore, when a horizontal well consists of multi-stage hydraulic fractures, the contact between the reservoir and the well is multiplied many times, thus leading to significant hydrocarbon production. However, only the porosity connected to the hydraulic fractures contributes to flow. Porosities that are not connected to the hydraulic fractures do not contribute to flow due to the very low reservoir permeability. This is the major difference between a conventional and unconventional reservoir. In a conventional reservoir, hydrocarbon flows from the reservoir to the hydraulic fracture by Darcy’s flow. In an unconventional reservoir, the flow of hydrocarbon to the hydraulic fracture is too slow to be effective. Therefore, only the porosity that is contacted to the hydraulic fractures will contribute to production. Figure 3 depicts the interaction between the hydraulic fracture and the shale porosity system.
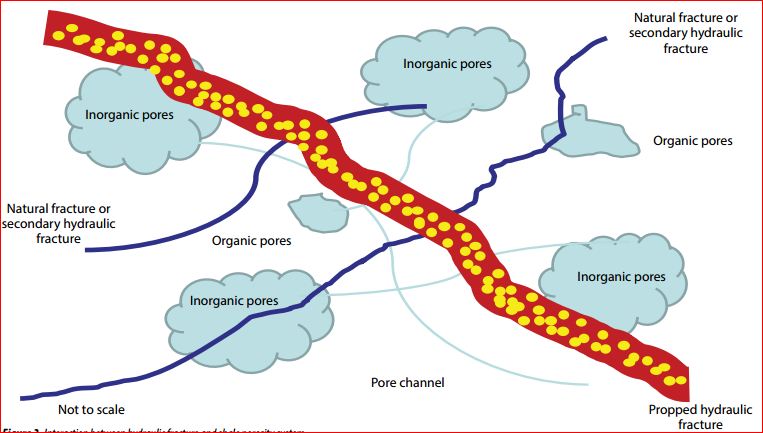 |
|
Figure 3. Interaction between hydraulic fracture and shale porosity system.
7. Maximising interaction between hydraulic fractures and shale porosities
One way to maximise the production of a shale reservoir is to increase the interconnectivity between the hydraulic fractures and the natural porosities of the reservoir. This can be done by reactivating and propping the natural fractures and the secondary hydraulic fractures.
Figure 4. Relative size of porosities in shale reservoirs.
- Connection between natural and hydraulic fractures
Field data suggest that some natural fractures are reactivated during the pumping stage of the hydraulic fracturing [7]. However, conventional proppants are too large (hundreds of microns) to enter the reactivated natural fractures (1 - 100µm). Consequently, when pumping stops, the natural fractures close and do not form conductive flow channels. In some cases, there may be some residual conductivity due to incomplete closing of the natural fractures.
- Connection between shale porosity system and hydraulic fractures
A hydraulic fracture extends from the wellbore to the shale reservoir when the injection pressure exceeds the fracture pressure of the shale. In conventional reservoirs, the fracture geometry is relatively simple and may be described as a planar fracture with well-defined fracture half length and fracture width. However, in very low permeability shale reservoirs, the fracture geometry becomes complex. Microseismic data suggest that the fractures are no longer planar, but have a rather complicated geometry [8] and resemble a network of complex fractures [9]. At present, microseismic is the tool most often used to map the fracture geometry. However, microseismic only measures locations in the reservoir where a shear failure occurs. It does not tell us whether these locations contribute to flow during production. Various authors have proposed different geometries of hydraulic fracture. However, the exact geometry of the hydraulic fracture is unknown to a large extent.
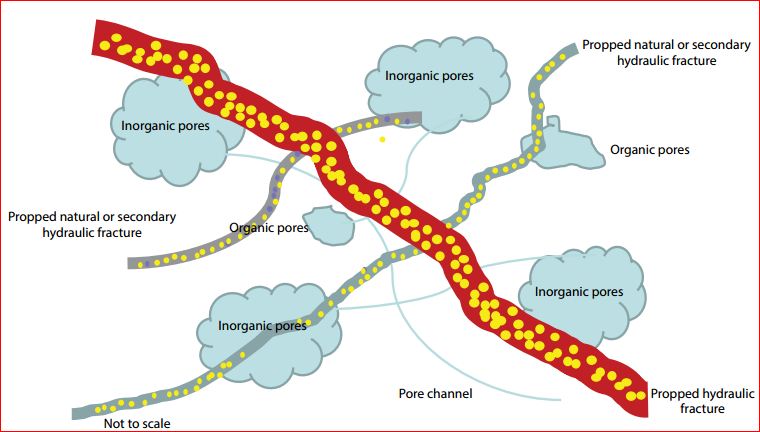 |
|
Figure 5. Increasing connectivity between hydraulic fractures and natural porosities by micro-sized proppants.
We postulate that the hydraulic fracture network has the following characteristics: (1) larger width, primary fractures extending from the horizontal well into the shale reservoir, (2) smaller width, secondary fractures extending from the primary fractures to other parts of the reservoir, and (3) connection between primary and secondary hydraulic fractures with natural fractures forming an extensive fracture network (Figure 3).
Figure 4 compares the relative size of porosities in a shale reservoir. Size of pores and pore channels ranges from 1 - 200nm. Natural fractures have an aperture between 1 - 100 microns. Primary hydraulic fractures have a width between 1 - 10mm, whereas secondary hydraulic fractures have a width between that of natural fractures and primary hydraulic fractures.
Conventional fracture designs assume a relatively simple planar fracture geometry and the treatment volume and flow rate are designed to create a fracture of pre- determined half length and fracture width. Furthermore, the fracture is propped with high-permeability proppant to resist crushing during production. Thus the fracture forms a highly conductive pathway for transport of hydrocarbon.
When applied to shale reservoirs, this type of design is inadequate because it assumes a single fracture width and uses a proppant size which is too big to enter into the secondary fractures or reactivated natural fractures. Since the secondary fractures and natural fractures are not propped, they contribute little to flow.
Our proposal is to inject micro-sized proppants small enough to enter the secondary and reactivated natural fractures. These propped fractures will greatly enlarge the fracture network and the stimulated reservoir volume (Figure 5).
8. Micro-sized proppants
In order to enter the reactivated natural fractures and secondary hydraulic fracture, micro-sized proppants should have diameter of 1 - 100 microns, i.e. 70 mesh or higher. Currently, proppants of this size range are not readily available.
- Laboratory study
So far, one operator and a service company have conducted a study on the use of micro-sized proppants for enhancing well production in Barnett shale [10, 11]. The micro-sized proppant used was a ceramic material having an average diameter of 30µm. Experiments were done with outcrop samples. Results show that the micro- sized proppant can increase the effective permeability of a micro fracture by as much as ten folds.
- Field pilot
A field pilot was conducted in eleven condensate rich gas wells in the Barnett shale in Wise County, Texas [10, 11]. Results show that incorporation of micro-sized proppant in the pad ahead of main hydraulic fracture treatment has resulted in a significant increase in well productivity over a 400-day period. Wells with micro-sized proppant exhibited 36 - 55% improvement in gas production and 23- 47% improvement in condensate production compared to those without the micro-sized proppant. However, Barnett shale is known to have well developed natural fractures, although most of them are sealed except the largest ones [7].
- Future research
Reported results on the use of micro-sized proppants to increase the effectiveness of hydraulic fracturing on Barnett shale have been encouraging. Future research should focus on other shale reservoirs with a less developed natural fracture network. It is also worthwhile to test the relative importance of propping the natural fractures versus secondary hydraulic fractures in various shale formations. Also, development of micro-sized proppants of various sizes, material and strength will be important for future application.
9. Conclusions
The following conclusions can be drawn from our study.
Shale reservoirs have a multi-porosity system consisting of organic pores, inorganic pores, pore channels and natural fractures. Due to the very small pore size (1 - 200nm), poor pore interconnectivity, and sealed natural fractures, shale reservoirs have very low permeability (< 0.001 - 0.01mD).
In shale reservoirs, horizontal wells by themselves are incapable of inducing flow. Multi-stage hydraulic fractures are needed to connect the horizontal wellbore to the shale porosity system. However, only those porosities which are connected to the hydraulic fractures will contribute to hydrocarbon production. Porosities not connected to the hydraulic fractures will not contribute to flow.
Hydraulic fractures in shale reservoirs consist of a complex network of fracture. We postulate that hydraulic fractures consist of primary and secondary fractures of different widths. Both types of fractures can connect with the natural fractures.
During hydraulic fracturing, some natural fractures are reactivated and are connected to the hydraulic fractures. However, since conventional proppants are too big to enter them and secondary hydraulic fractures, they close when pumping stops and do not contribute significantly to hydrocarbon production.
One way to maximise well productivity is to inject micro-sized proppants (1 - 100 microns) into the secondary hydraulic fractures and the reactivated natural fractures, thereby extending the fracture network. This will also increase the stimulated reservoir volume.
Recent laboratory results have shown that a micro- sized ceramic proppant is effective in enhancing the permeability of micro-fracture in Barnett shale. In addition, an eleven-well pilot test showed that incorporation of the micro-sized proppant in the pad of fracture treatment increased the productivity of these wells by up to 50%.
Future research is needed to study the use of micro- sized proppants for productivity enhancement in other shale formations. Research is needed to tailor the size, type and strength of micro-sized proppants for particular shale formations.
References
1. Brian J.Davis, Russell Maharidge, Joel Walls. Chapter 10: Laboratory tests and considerations to complement the overall reservoir understanding. In “Unconventional oil and gas resources exploitation and development”. CRC Press.
2. Abdus Satter, Ghulam M.Iqbal. Reservoir engineering: The fundamentals, simulation, and management of conventional and unconventional recoveries. Elsevier. 2016.
3. Matt W.Bratovich, Frank Walles. Formation evaluation and reservoir characterization of source rock reservoirs. In “Unconventional Oil and Gas Resources Exploitation and Development”. CRC Press. 2016.
4. Gareth Chalmers, Ian M.Power, R.Marc Bustin. Characterization of gas shale pore systems by porosimetry, pycnometry, surface area, and field emission scanning electron microscopy/transmission electron microscopy image analyses: Examples from the Barnett, Woodford, Haynesville, Marcellus, and Doig Units. AAPG Bulletin. 2012; 96(6): p. 1099 - 1119.
5. Caineng Zou. Unconventional petroleum geology. Elsevier. 2017.
6. Philip H.Nelson. Pore-throat sizes in sandstones, tight sandstones and shales. American Association of Petroleum Geologists Bulletin. 2009; 93(3): p. 329 - 340.
7. Julia Fiona Wells Gale, Robert M.Reed, Jon Holder. Natural fractures in the Barnett shale and their importance for hydraulic fracture treatments. American Association of Petroleum Geologists Bulletin. 2007; 91(4): p. 603 - 622.
8. Koras Shah, Robert Frank Shelley, Deepak Gusain, Lyle V.Lehman, Amir Mohammadnejad, Matthew T.Conway. Development of a Brittle shale fracture network model. SPE 163829. SPE Hydraulic Fracturing Technology Conference, Woodlands, Texas, USA. 4 - 6 February, 2013.
9. Martin Rylance, Tony Martin. Flushing away the profit: The potential misapplication of unconventional fracturing technology on a global scale. SPE 170773. SPE Annual Technical Conference and Exhibition, Amsterdam, the Netherlands. 27 - 29 October, 2014.
10. Jeff Dahl, Philip Nguyen, Ron Dusterhoft, James Calvin, Shameem Siddiqui. Application of micro-proppant to enhance well production in unconventional reservoirs: Laboratory and field results. SPE 174060. SPE Western Regional Meeting, Garden Grove, California, USA. 27 - 30 April, 2015.
11. Jeff Dahl, James Calvin, Shameem Siddiqui, Philip Nguyen, Ron Dusterhoft, Eric Holderby, Bill Johnson. Application of micro-proppant in liquids-rich, unconventional reservoirs to improve well production: Laboratory results, field results, and numerical simulations. SPE 177663. Abu Dhabi International Petroleum Exhibition and Conference, Abu Dhabi, UAE. 9 - 12 November, 2015.