Sources, mechanism and prediction method of scale formation in oil production in Vietnam
11:14 |
09/11/2018
Lượt xem:
6890
Kieu Anh Trung (1), Nguyen Minh Quy (1), Hoang Long (1), Truong Van Dung (1), Le Thi Thu Huong (1), Pham Thi Thuy(2), Vu Huu Huy(2)
1Vietnam Petroleum Institute, 2Hoan Vu Joint Operating Company Email: trungka.epc@vpi.pvn.vn
Summary
This paper presents the typical sources and mechanism of scale formation in oil production in Vietnam. A workflow of prediction method to find scale formation during oil production was presented. The main sources of scale problem in oil production are incompatible mixing between different water sources and the change of pressure/temperature along the production system. A case study using the prediction method was also introduced in the article. The decrease of productivity index of the observed well can be caused by the dominant build-up of scale at downhole. Based on the mechanism of scale formation, a suitable scale control strategy was given.
Key words: Scale formation, scale prediction, water production, productivity index.
1. Introduction
Today, the problem of inorganic scaling becomes more and more serious in many oil fields in Vietnam, such as Bach Ho, Te Giac Trang, Ca Ngu Vang, Rang Dong, and Su Tu Vang. Few production problems strike fear into the hearts of engineers the way scale can. Scale is an assemblage of deposits that cake perforations, casing, production tubing, valves, pumps and downhole completion equipment, thereby clogging the wellbore and preventing fluid flow. Scale, just like the scale found in home plumbing or tea kettles, can be deposited all along water paths from injectors through the reservoir to surface equipment. Most scales found in oil fields in Vietnam form either by direct precipitation from the water that occurs naturally in reservoir rocks, or as a result of produced water becoming oversaturated with scale components when two incompatible waters meet downhole. Whenever an oil or gas well produces water, or water injection is used to enhance recovery, there is the possibility that scale will form.
The buildup of scale inside wellbore and production equipment (both subsurface and surface) has badly affected oil production efficiency, causing millions of dollars in damage every year. Scale can develop in the formation pores near the wellbore and reduce formation porosity and permeability. It can block the flow by clogging perforations or forming a thick lining in production tubing. It can also coat and damage downhole completion equipment, such as safety valves and gas- lift mandrels. The productivity index can fail half in just several weeks and therefore that requires serious study of scale problem to find out the suitable cures [1].
In this article, we present the mechanism and source of scale buildup during oil production. Knowing the conditions that lead to scaling and when and where it occurs helps in understanding how to prevent and/or remove scale to restore long-term well productivity. A scaling assessment job that has been done at an offshore oil field by the Vietnam Petroleum Institute (VPI) and Hoan Vu Joint Operating Company (Hoan Vu JOC) will also be presented in this paper as a case study.
2. Main mechanism of scale in oil production
The production of water is a natural consequence of the production of oil and gas from subterranean reservoirs. Water that is present in these reservoirs contains dissolved salts and dissolved gases. Under certain conditions these salts precipitate and become scale deposits [1]. It is not possible to produce oil and gas for any significant time before water is also produced. The water/oil boundary will rise over time and channelling from water permeable zones will cause increased water to be produced. Scales are formed due to precipitation and crystal growth at the water surface. Scale begins to form when the state of any natural fluid is perturbed such that the solubility limit for one or more components is exceeded. Mineral solubility itself has a complicated dependence on temperature and pressure.
There are two basic mechanisms by which scales are formed in the petroleum reservoir [2]:
- Autoscaling
A reservoir fluid experiences changes in temperature and pressure as it is produced. If such changes take the fluid composition beyond the solubility limit for a mineral, it will precipitate as scale, this phenomenon is called autoscaling or self-scaling. Sulfate and carbonate scales can precipitate as a result of pressure changes within the wellbore or at any restriction downhole. Sodium chloride scale (halite) forms in a similar way from highly saline brines undergoing large temperature drops.
Another serious problem occurs when carbonate scales precipitate from produced fluids containing acid gases. Reduction in pressure during production outgases the fluid, which raises pH and causes scale deposition. The deposition of carbonate can extend from the near-wellbore matrix, along tubing and into surface equipment as the produced water continuously changes in pressure and temperature. For carbonate scales, temperature effects often work against pressure effects. For example, the pressure drop at the point of entry into the wellbore can lead to matrix scale. As the fluid progresses up the tubing to surface temperatures and wellhead pressure, the resulting temperature drop may override the pressure effect, reducing scale formation in the tubing. On the other hand, subsequent release of pressure from the wellhead to the surface can lead to massive deposits of scale in surface equipment and tubing.
- Incompatible mixing
Mixing incompatible injection and formation waters can cause scale formation. Seawater is often injected into reservoirs during secondary and enhanced-recovery water flooding operations. Seawater is typically rich in SO 2- anions with concentrations often above 2,000mg/L (0.02ppg), while formation waters contain divalent cations Ca2+ and Ba2+. Fluid mixing in the near-wellbore matrix generally produces new fluids with combined ion concentrations that are above the solubility limits for sulfate minerals. Calcium sulfate (CaSO4) scale forms in limestone formations, and barium sulfate (BaSO ) and strontium sulfate (SrSO ) scales form in sandstone formations. If these scales form in the formation, they are difficult to remove chemically and impossible to remove mechanically. Incompatible water mixing can also occur in tubing, producing scales that are accessible to both chemical and mechanical removal.
- Forming scale
Although the driving force for scale formation may be a temperature or pressure change, out-gassing, a pH shift, or contact with incompatible water, many produced waters that have become oversaturated and scale-prone do not always produce scale. In order for a scale to form it must grow from solution. The first development within a saturated fluid is a formation of unstable clusters of atoms, a process called homogeneous nucleation. The atom clusters form small seed crystals triggered by local fluctuations in the equilibrium ion concentration in supersaturated solutions. The seed crystals subsequently grow by ions adsorbing onto imperfections on the crystal surfaces extending the crystal size. The energy for seed crystal growth is driven by a reduction in the surface free energy of the crystal, which decreases rapidly with increasing radius after a critical radius is exceeded. This implies that large crystals favour continuing crystal growth, and also implies that small seed crystals may rediscover. Thus, given a large enough degree of supersaturation, the formation of any seed crystal will encourage an increase in the growth of scale deposits. The seed crystal, in effect, is a catalyst for scale formation. Crystal growth also tends to initiate on a pre-existing fluid-boundary surface, a process called heterogeneous nucleation. Heterogeneous nucleation sites include surface defects such as pipe surface roughness or perforations in production liners, or even joints and seams in tubing and pipelines. A high degree of turbulence can also catalyse scale deposition. Thus, the accumulation of scale can occur at the position of the bubble point pressure in the flowing system. This explains why scale deposits rapidly build on downhole completion equipment.
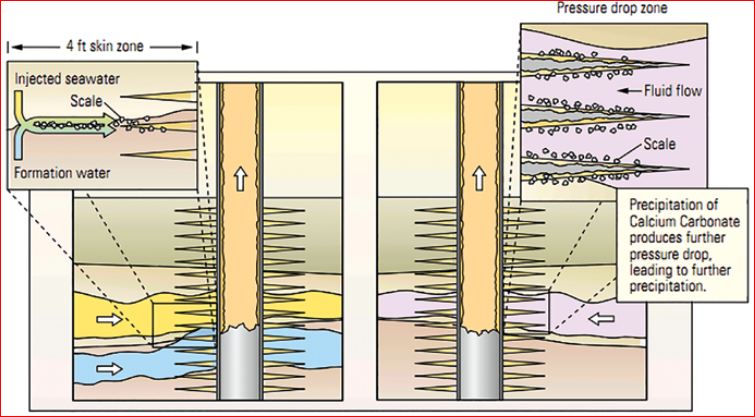 |
|
Figure 1. Two basic mechanisms of scales formed in the petroleum reservoir [1].
Table 1. The location and composition of the scale deposit
(a) (b)
Figure 2. Inorganic scale formed in the production system, Vietnam’s oil field (a: at tubular; b: at choke valve).
- Identifying scale
Identifying the location and composition of the scale deposit is the first step in designing a cost-effective remediation programme (Table 1).
3. Scale control method to reduce problem cost by scale formation
The direct cost of removing scale from one well can be as high as USD 2.5 milion, and the cost of deferred production even higher [3]. Just as prevention is better than cure in medical practice, keeping producing wells healthy is ultimately the most efficient way to produce hydrocarbons. In most cases, scale control method starts with scale prediction (forecast) and needs to be updated regularly based upon surveillance. This method will balance preventive measures and remediation (removal method). From the results of prediction of scale, a suitable scale control strategy will be described including prevention and remediation methods.
(a) Prevention
Choosing suitable chemical inhibition - continuous or periodic squeezes;
In most cases, scale prevention through chemical inhibition is the preferred method of maintaining well productivity. Inhibition technique can range from basic dilution method, to the most advanced and cost-effective method of threshold scale inhibitor.
(b) Remediation: Scale removal, either chemical or physical.
Scale removal techniques must be quick, non-damaging to the wellbore, tubing or formation environment, and effective at preventing reprecipitation. The best scale-removal technique depends on knowing the type and quantity of scale, and its physical composition or texture. A poor choice of removal method can actually promote the rapid recurrence of scale.
The following workflow is presented to summarise the scale control method:
4. Case study using scale prediction meth- od done by VPI and Hoan Vu JOC
The A oil field has produced oil from fractured basement reservoir with 4 produced wells, in which 1P-SCL has the highest production rate. However, the well productivity index (PI) of 1P-SCL significantly dropped from 6.2 to 3.0bpd/psi after just 2 years of first oil during a short period (several weeks).The pressure survey is carried out in an yearly basis to determine reservoir pressure and well PI. The skin factor could not be estimated in the 1P-SCL well due to wellbore storage effects. It is essential to quantify and characterise the scale formation downhole in 1P-SCL, focusing on near-wellbore area where pressure drop takes place in flowing condition. Therefore, the best strategy in combating losses experienced due to scaling is periodical prevention of scale formation. VPI has conducted this study for Hoan Vu JOC to propose the suitable method with the scale problem of 1P-SCL in the A oil field.
All data relating to drilling, completion and operation phases of the 1P-SCL well were gathered and reviewed. Current production water of 1P-SCL was also sampled and analysed. To support for this study, many experimental tests and analyses were conducted including the filtration membrane tests, self-scaling tests and incompatible mixing test using HTHP (high temperature, high pressure) laboratory system. Additionally, a computational modelling has also been undertaken with the support from the latest version of ScaleChem software to evaluate the scale tendency and scale amount.
4.1. Production water composition
Initially, the reservoir pressure decreased continuously with very low water cut < 3%. The reservoir pressure decreased from around 7,200psia to 5,600psia after 5 years of operation. It means that there has been no aquifer support for the reservoir pressure. Besides, the composition of 1P-SCL water at the beginning was very rich in Cl-, then the Cl- content decreased right after the water breakthrough. The 1P-SCl has its production target at basement reservoir (more than 4,000mD), therefore, during the drilling phase, a very high density drilling mud was used. This drilling mud was CaCl2 with very high content of Cl- (200,000mg/L). Generally, in the basement of the referent oil field in the South East offshore Vietnam, the connate formation water (if available) may be immigrated from above reservoir (Oligocene) with low total dissolved solid (TDS) value (< 40,000mg/L). However, the TDS value of 1P-SCL production water is quite high (> 90,000mg/L). Hence, the production water of 1P-SCL can be considered as drilling mud losses only (at the initial time of production) and the mixture of drilling mud losses with injection water at a later phase.
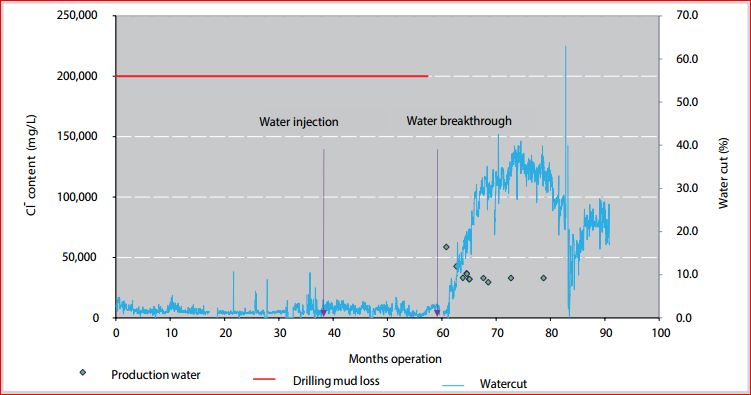 |
|
Figure 3. Cl- content and water cut in historical production water composition of 1P-SCL well.
Figure 4. Scale scenario at downhole of 1P-SCL [6].
After water breakthrough occurred the water rate and cut increased within a few months to approximately 1,500 barrels/dayand 38% respectively. Seawaterbreakthrough, from injection well to 1P-SCL was marked also by a rapid decline in Cl- in production water (Figure 3) and approximately coincided with the start of water production in this well suggesting that breakthrough occurred within the oil-leg (Cl- is used as a conservative natural tracer [4]). Before water breakthrough, around 105,000 barrels of drilling losses (brine) were recovered. The remaining approximate 25,000 barrels of brine could be mixed with injection water during several months leading to the decrease of Cl- content in production water. The Cl- content sharply decreased and then kept stably at around 30,000mg/L during 15 months. By calculation, the mixing ratio at this period is about 95% of injection water. This ratio is acceptable if compared to the remaining drilling mud loss out of total production water.
4.2. Scale scenarios at 1P-SCL well
By analysis of the water composition of production water and the drilling mud loss, the scale may form at downhole of 1P-SCL according to 2 scenarios:
- The incompatible mixing between injection water and drilling mud loss at the time of water breakthrough. The contact of seawater rich in SO 2- with drilling mud loss (CaCl2) will form CaSO4, an inorganic salt with very low solubility at high pressure and high temperature [5]:
Ca2+ + SO 2- → CaSO ↓
- The change of pressure from reservoir to downhole. As these fluids will experience drop in pressure, CO2 leaving leading the formation of CaCO3:
Figure 5. Scale tendency at the different mixing ratio at downhole of 1P-SCL.
Table 2. Scale amount formed at downhole of 1P-SCL by ScaleChem
Figure 6. High pressure, high temperature laboratory system.
Table 3. Composition of injection water and drilling mud loss for the test
4.3. Prediction of scale tendency at downhole of 1P-SCL by ScaleChem software
Desktop scale study is simulated and predicted the scaling tendency and severity for oil production system including auto- scaling at subsurface and surface facilities and incompatible mixing between different sources of water by ScaleChem software. The results of the scaling prediction are frequently used to determine whether scaling is likely to occur and the scale species likely to be formed at subsurface/surface condition.
Table 4. Dynamic data of 1P-SCL for the test
Figure 7. SEM result of solid scale collected from laboratory test.
Figure 8. XRD results of solid scale collected from laboratory test.
All analytical results obtained from laboratory tests will be used for computation and also used as input data for software.
The possibility and severity of scale formation will be calculated and simulated using ScalingChem® software. ScalingChem® software predicts mineral scaling potentials of 80+ solids for virtually any oil and gas well and processing facility as CaSO4, CaCO3, CaSO4, CaSO4.2H2O, BaSO4, BaCO3, Fe(OH)3, Fe2O3, CrSO4, CrCO3,NaCl, plus of scales of F-, OH-, Cl-, Br-, S2-, oxides and acids. The programme has advanced the simulation and prediction accuracy for sulphate, carbonate and iron scales [7]. The programme took into consideration a range of factors governing scale precipitation such as activity coefficients, thermodynamics, common ion and ion pair effects, varying pH, and in some cases, the influence of oil and gas compositions, GWR and OWR for accuracy predicting of sulphate and carbonate scale deposition.
• Desktop results:
The composition of injection water, drilling mud loss and the dynamic data at downhole of 1P-SCL were used as input data of ScaleChem software. The results of incompatible mixing are given at Figure 5. Thereby, at mixing ratio of 95% injection water, two inorganic materials were found as CaCO3 (calcite) and CaSO4 (anhydrite). When the fluid comes out to downhole of 1P-SCL, the pressure drop leads to the formation of CaCO3 scale. The detail calculation of scale amount was summarised in Table 2.
4.4. Laboratoryteststosimulatetheformationofscaleatdownhole
Scale prediction tests were conducted to confirm the results of the computer scale predictions and capture the types of scale precipitation at downhole of 1P-SCL due to mixing incompatibility or change of pressure/temperature condition. Using the high pressure, high temperature laboratory system to assess the scaling risk associated with several of pressure at different temperatures of oil process system and interaction between water sources.
The mixing ratio between 2 sources is 95-5 as calculation above. The solid scale obtained in the tests on the membrane will be analysed by SEM-EDS and XRD to determine the type of scale. The amount of scale is also determined.
• Laboratory test results:
The SEM image shows clearly that there are two types of crystal formed in the test, one of needle-shaped crystal and the other of powder shaped and smaller.
EDS results (element composition analysis) on the needle-shaped crystal show the elements of calcium, sulphur and oxygen account for CaSO4 and on the powder-shaped crystal show the elements of calcium, carbon and oxygen account for CaCO3.
The XRD results also illustrate two types of peak of two crystals: anhydrite (CaSO4) and calcite (CaCO3) with weight fraction of 96% and 4% accordingly.
4.5.Evaluation and recommendation
The desktop work results show abundance of CaSO4 scale with some CaCO3 that can be formed by the incompatible mixing between injection water and drilling mud brine and by the pressure drop. As per the computational modelling results, the worst case predicts a total amount of scale precipitation of approximately 2,373mg/L in near well-bore/downhole area. Using the laboratory test, a solid amount of around 2,089mg/L was collected in the test conducted under near-wellbore/downhole condition. Hence, both results of laboratory and modelling are in the same range.
Through reviewing the data and evaluating the laboratory test results as well as software modelling results, the major cause of PI drop of the 1P-SCL well is recognised. The results show that the incompatibility between calcium-based brine drilling mud fluid and injection water containing high sulfate causes scale precipitation. However, at the moment of water sampling, the existence of drilling mud in the production fluid is almost negligible, therefore this decrease of PI caused by CaSO4 scale will not continue.
To restore the PI, the chelating-based chemical (such as EDTA) should be applied for the downhole of 1P-SCL well. The following reactions illustrate the mechanism of dissolver performance [3]:
CaSO + Na EDTA → CaNa EDTA + Na SO
Generally, once the scale is dissolved through chelation, there is no re-precipitation. Stable at temperatures up to 250oC, the chelating agent is a low toxicity scale dissolver that is effectively noncorrosive on most steels- making the treatment extremely safe.
5. Conclusions
• Scale monitoring plan and prediction of the scale formation should be carried out frequently to minimise the bad effect of inorganic scale to oil production.
• The incompatibility between injection water (seawater) and formation water or drilling mud brine needs to be studied prior to water injection or EOR application.
• The selection of scale prevention and remedial method must be based on the mechanism and source of scale formation.
• High density calcium-based brine during drilling and completion phase should be used with special caution to minimise the incompatibility with injection water containing high sulfate content.
References
1. Mike Crabtree, David Eslinger, Phil Fletcher, Ashley Johnson, George King. Fighting scale: Removal and prevention. The BP Technology Magazine. 1998.
2. Guihong Pei, Chunyang Wang, Lili Liu. Experimental study on the cause of inorganic scale formation in the water injection pipeline of Tarim oilfield. Journal of Chemistry. 2014.
3. J.Moghadasi,H.Müller-Steinhagen,M.Jamialahmadi, A.Sharif. Scale deposition in porous media and their removal by EDTA injection. Engineering Conferences International Symposium Series: Proceedings of 7th International Conference on Heat Exchanger Fouling and Cleaning - Challenges and Opportunities, Tomar, Portugal. 1 - 6 July, 2007.
4. Francisco J.Alcalá, Emilio Custodio. Using the Cl/Br ratio as a tracer to identify the origin of salinity in aquifers in Spain and Portugal. Journal of Hydrology. 2008; 359(1 - 2): p. 189 - 207.
5. Amer Badr Bin Merdhah, Abu Azam Mohd Yassin. Study of scale formation in oil reservoir during water injection - A review. Marine Science & Technology Seminar, Kuala Lumpur, Malaysia. 22 - 23 February, 2007.
6. Amer Badr Bin Merdhah, Abu Azam Mohd Yassin. Scale formation in oil reservoir during water injection at high-salinity formation water. Journal of Applied Sciences. 2007; 7(21): p. 3198 - 3207.
7. M.Amiri, J.Moghadasi. Prediction the amount of barium sulfate scale formation in Siri oilfield using OLI ScaleChem software. Asian Journal of Scientific Research. 2010; 3(4): p. 230 - 239.
Bình luận